
Outline of Our Research Program
The current focus of our group is to synthesize and study magnetic and magnetotransport properties of various quantum materials. Materials include Correlated topological materials such Dirac, Weyl semi(metals) and flat band systems, chiral magnets, and novel antiferromagnets such as altermagnets.
Research Motivation
Over the past few decades, the forefront of hard condensed matter physics has undergone a quiet revolution, ushering in the age of topological quantum materials. The physics of topological materials is typically well described by band theory in systems of non-interacting fermions. In contrast, several of the most fascinating effects in condensed matter physics exist entirely due to electron-electron interactions. Some of the examples are unconventional superconductivity, heavy fermions, the Kondo effect, and the Mott transition. Therefore, there is currently a great deal of interest to know what the effect of interactions in these topological materials might be.
Advancement of the physics of strongly correlated materials have mostly been prompted through the discovery of new materials. Being a material synthesis group, we see an opportunity here to discover new phenomena in the correlated topological materials by synthesizing them and measuring their physical properties. We are interested in the fundamental understanding of the following open questions.
1) Does the topological band structure survive in presence of electron-electron interactions?
2) Can the electron-electron interactions turn a topologically trivial ground state into a non-trivial one?
3) What exotic states of matter emerge when the non-trivial band topology competes with strong electron-electron correlations?
Answers to these questions not only advance our understanding of the physics of correlated topological materials but also lay a foundation for future technologies based on the effect of such interactions such as smaller and faster electronic devices, quantum computers, etc.
Research Highlights/Press Coverage
2021: Ghimire awarded NSF CAREER Award
2020: Mason, NIST, ANL and ORNL collaboration discovers a new topological magnetic state
2018: Scientists find unusual behavior in topological material
Recent Projects
Magnetism and Fermiology of Kagome Magnet YMn6Sn4Ge2
Preprint: arXiv:2304.11502
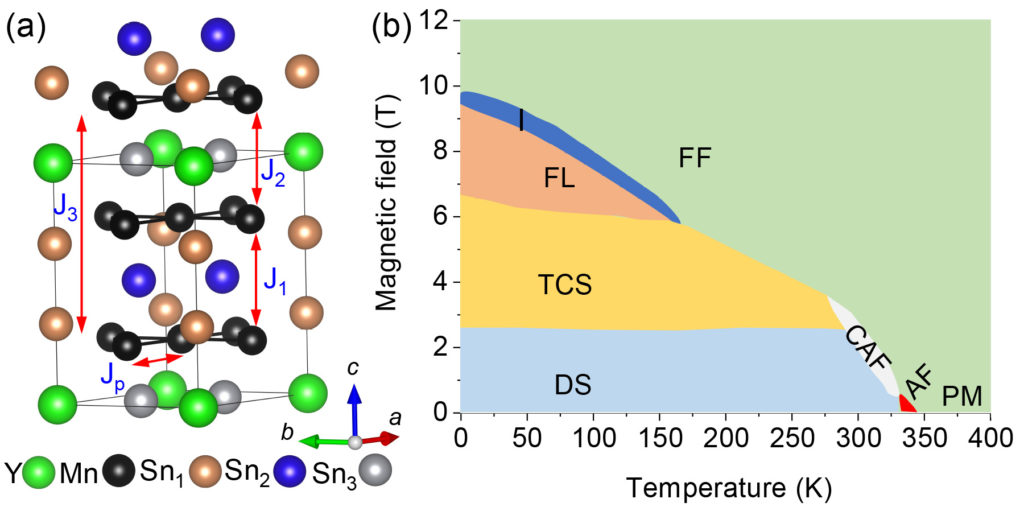
Kagome lattice magnets are an interesting class of materials as they can host topological properties in their magnetic and electronic structures. YMn6Sn6 is one such compound in which a series of competing magnetic phases is stabilized by an applied magnetic field, and both an enigmatic topological Hall effect and a Dirac crossing close to the Fermi energy have been realized. This material also shows a magnetization-induced Lifshitz transition and evidence of a unique charge spin coupling in one of the magnetic phases, namely the fan-like phase. Tuning the magnetism, and thus the interplay with the electronic states, opens new avenues for precise control of these novel properties. Here, we demonstrate the extreme sensitivity of the magnetic phases in YMn6Sn4Ge2 through the investigation of structural, magnetic, and transport properties. The high sensitivity to small doping provides great potential for engineering the magnetic phases and associated electronic properties in this family of rare-earth kagome magnets.
Origin of spin reorientation and intrinsic anomalous Hall effect in the kagome ferrimagnet TbMn6Sn6.
Preprint: arXiv:2203.17246
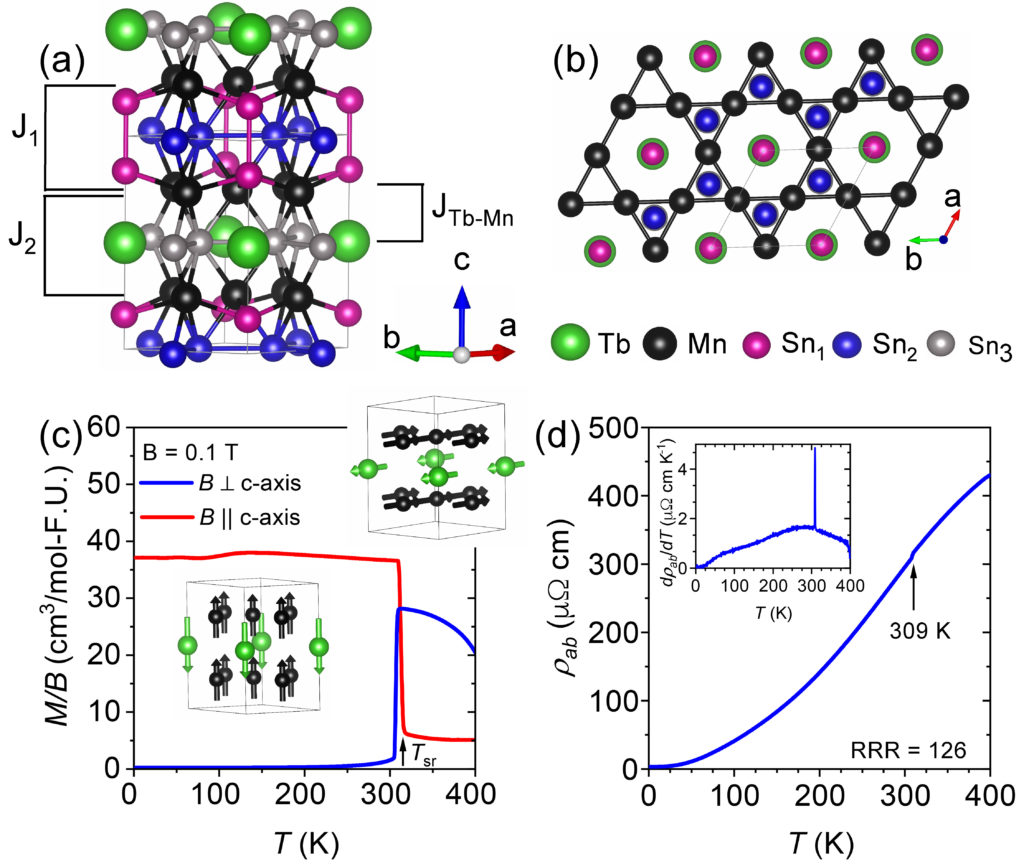
TbMn6Sn6 has attracted a lot of recent interest for a variety of reasons, most importantly, because of the hypothesis that it may support quantum-limit Chern topological magnetism, derived from the kagome geometry. Besides, TbMn6Sn6 features a highly unusual magnetic reorientation transition about 100 K below the Curie point, whereby all spins in the system, remaining collinear, rotate by 90∘. In this work, we address both issues combining experiment, mean-field theory and first-principle calculations. Both magnetic reorientation and the unusual temperature dependence of the anomalous Hall conductivity (AHC) find quantitative explanation in the fact that Mn and Tb, by virtue of the Mermin-Wagner theorem, have very different spin dynamics, with Tb spins experiencing much more rapid fluctuation. We were able to cleanly extract the intrinsic AHC from our experiment, and calculated the same microscopically, with good semiquantitative agreement. We have identified the points in the band structure responsible for the AHC and showed that they are not the kagome-derived Dirac points at the K-corner of the Brillouin zone, as conjectured previously.
CoTe2: A quantum critical Dirac metal with strong spin fluctuations
Advanced Materials 2300640 (2023) (open access)
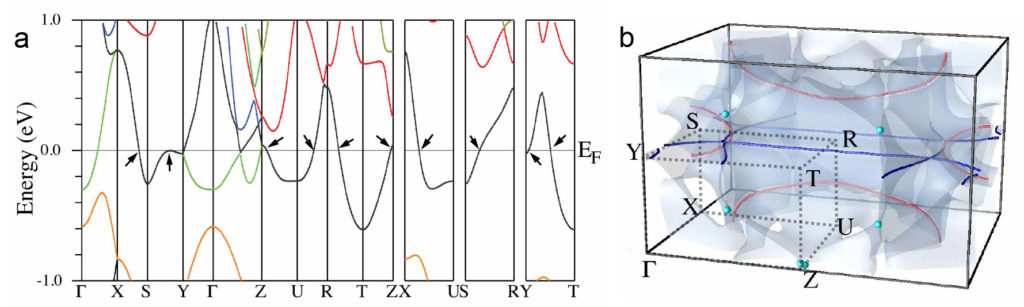
Quantum critical points separating weak ferromagnetic and paramagnetic phases trigger many novel phenomena. Dynamical spin fluctuations not only suppress the long-range order, but can also lead to unusual transport and even superconductivity. Combining quantum criticality with topological electronic properties presents a rare and unique opportunity. Here, by means of ab initio calculations and magnetic, thermal, and transport measurements, it is shown that the orthorhombic CoTe2 is close to ferromagnetism, which appears suppressed by spin fluctuations. Calculations and transport measurements reveal nodal Dirac lines, making it a rare combination of proximity to quantum criticality and Dirac topology.
LiMo8O10: Polar Crystal Structure with Infinite Edge-Sharing Molybdenum Octahedra
Inorganic Chemistry 2022, 61, 35, 13924–13932
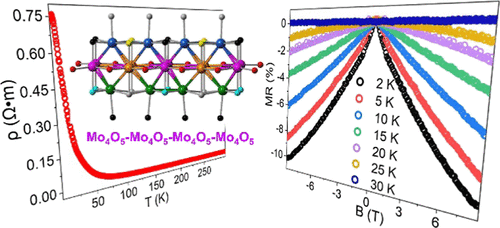
Polycrystalline LiMo8O10 was prepared in a sealed Mo crucible at 1380 °C for 48 h using the conventional high-temperature solid-state method. The polar tetragonal crystal structure (space group I41md) is confirmed based on the Rietveld refinement of powder neutron diffraction and 7Li/6Li solid-state NMR. The crystal structure features infinite chains of Mo4O5 (i.e. Mo2Mo4/2O6/2O6/3) as a repeat unit containing edge-sharing Mo6 octahedra with strong Mo-Mo metal bonding along the chain. X-ray absorption near-edge spectroscopy of the Mo-L3 edge is consistent with the formal Mo valence/configuration. Magnetic measurements reveal that LiMo8O10 is paramagnetic down to 1.8 K. Temperature-dependent resistivity [ρ(T)] measurement indicates a semiconducting behavior that can be fitted with Mott’s variable range hopping conduction mechanism in the temperature range of 215 and 45 K. The ρ(T) curve exhibits an exponential increase below 5 K with a large ratio of / = 435. LiMo8O10 shows a negative field-dependent magnetoresistance between 2 and 25 K. Heat capacity measurement fitted with the modified Debye model yields the Debye temperature of 365 K.
Ultralow Lattice Thermal Conductivity in Metastable Ag2GeS3 Revealed by a Combined Experimental and Theoretical Study
Chemistry of Materials 2022, 34, 14, 6420–6430.
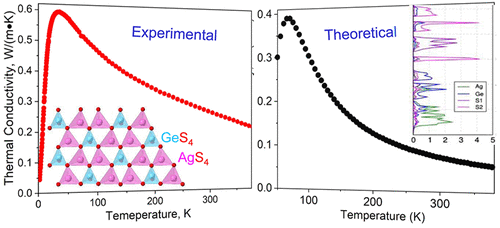
Metastable polycrystalline Ag2GeS3 compound was prepared at 1000 °C with binary Ag2S and GeS2 as starting materials. At room temperature, Ag2GeS3 was determined to adopt a polar orthorhombic crystal structure (space group Cmc21) based on the Rietveld refinement of synchrotron X-ray diffraction data. The crystal structure consists of layers of distorted AgS4 and GeS4 tetrahedra stacking along the crystallographic c axis. UV-vis diffuse reflectance spectra identify Ag2GeS3 as a semiconductor with an optical indirect band gap of 2.04 eV. Thermal analysis inside a sealed tube indicates that Ag2GeS3 undergoes several phase transitions but reforms upon cooling after heating to 1000 °C. Thermoelectric conductivity measurements show that Ag2GeS3 exhibits glass-like ultralow lattice thermal conductivity of 0.26 W/m∙K at 300 K and 0.22 W/m∙K at 380 K. Theoretical calculation of lattice thermal conductivity based on density functional theory (DFT) using the modified Debye-Callaway model shows a good qualitative agreement with experimental results between 50 K and 400 K. A combination of zig-zag atomic arrangement that decorate the crystal structure, electronic structure features dominated by the hybridization of Ag-S bonds near the Fermi energy, and nearly flat or dispersion-less low energy optical phonon branches where Ag acts as the rattler atom provides plausible reasons for the observed ultralow lattice thermal conductivity.
Visualizing the out-of-plane electronic dispersions in an intercalated transition metal dichalcogenide
Physical Review B, 105, L121107 (2022)
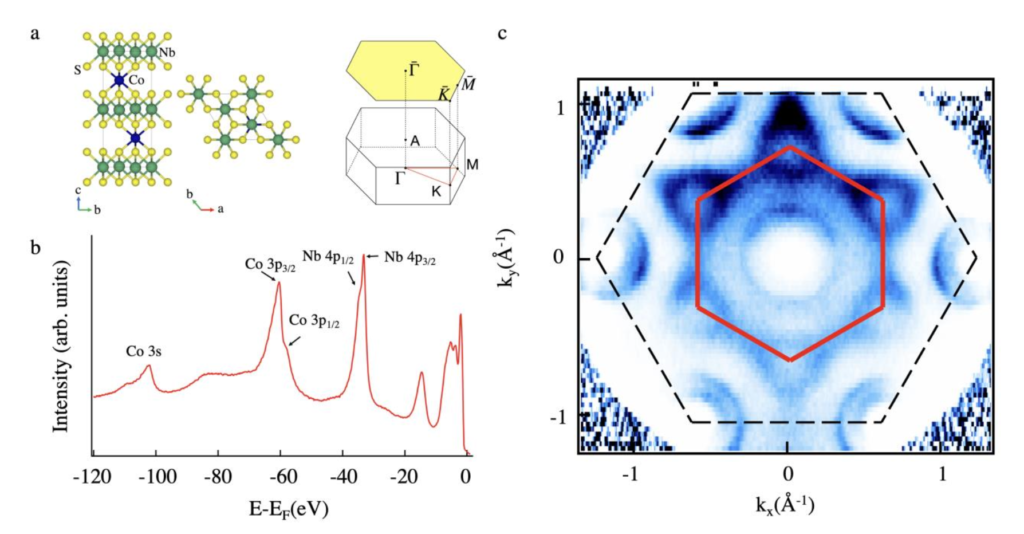
Layered transition metal dichalcogenides have rich phase diagram and they feature two dimensionality on numerous physical properties. Co1/3NbS2 is one of the newest members of this family where Co atoms are intercalated into the Van der Waals gaps between NbS2 layers. We study the three-dimensional electronic band structure of Co1/3NbS2 using both surface and bulk sensitive angle-resolved photoemission spectroscopy. We show that the electronic bands don’t fit into the rigid-band-shift picture after the Co intercalation. Instead, Co1/3NbS2 displays a different orbital character near the Fermi level compared to the pristine NbS2 compound and has a clear band dispersion in kz direction despite its layered structure. Our photoemission study demonstrates the out-of-plane electronic correlations introduced by the Co intercalation, thus offering a new perspective on this compound. Finally, we propose how Fermi level tuning could lead to exotic phases such as spin density wave instability.
Magnetization-driven Lifshitz phase transition and charge-spin coupling in the kagome metal YMn6Sn6
Communications Physics 5, 58 (2022)
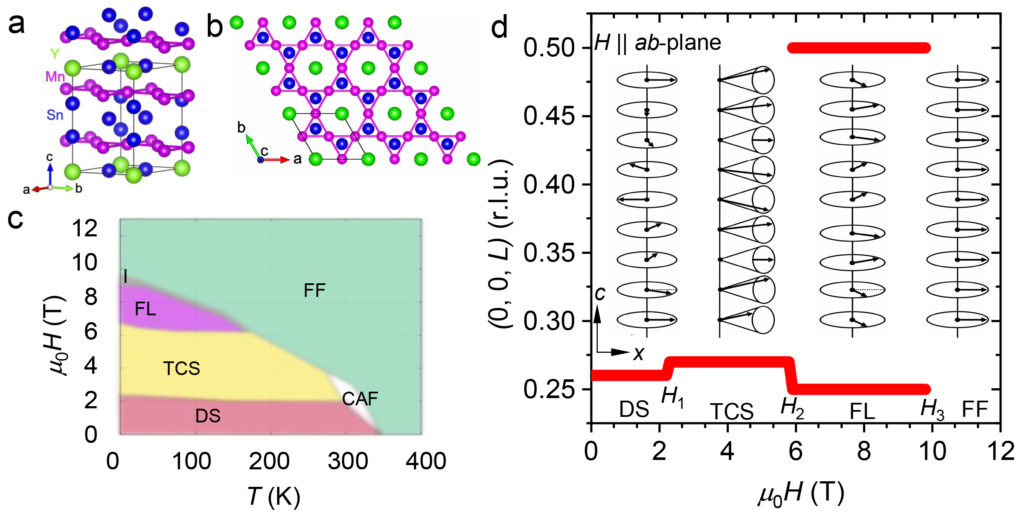
The Fermi surface (FS) is essential for understanding the properties of metals. It can change under both conventional symmetry-breaking phase transitions and Lifshitz transitions (LTs), where the FS, but not the crystal symmetry, changes abruptly. Magnetic phase transitions involving uniformly rotating spin textures are conventional in nature, requiring strong spinorbit coupling (SOC) to influence the FS topology and generate measurable properties. LTs driven by a continuously varying magnetization are rarely discussed. Here we present two such manifestations in the magnetotransport of the kagome magnet YMn6Sn6: one caused by changes in the magnetic structure and another by a magnetization-driven LT. The former yields a 10% magnetoresistance enhancement without a strong SOC, while the latter a 45% reduction in the resistivity. These phenomena offer a unique view into the interplay of magnetism and electronic topology, and for understanding the rare-earth counterparts, such as TbMn6Sn6, recently shown to harbor correlated topological physics.
Iridate Li8IrO6: An Antiferromagnetic Insulator
Inorg. Chem. 2021, 60, 17201−17211

A polycrystalline iridate Li8IrO6 material was prepared via heating Li2O and IrO2 starting materials in a sealed quartz tube at 650 °C for 48 h. The structure was determined from Rietveld refinement of room-temperature powder neutron diffraction data. Li8IrO6 adopts the nonpolar space group R3̅ with Li atoms occupying the tetrahedral and octahedral sites, which is supported by the electron diffraction and solid-state 7Li NMR. This results in a crystal structure consisting of LiO4 tetrahedral layers alternating with mixed IrO6 and LiO6 octahedral layers along the crystallographic c-axis. The +4 oxidation state of Ir4+ was confirmed by near-edge X-ray absorption spectroscopy. An in situ synchrotron X-ray diffraction study of Li8IrO6indicates that the sample is stable up to 1000 °C and exhibits no structural transitions. Magnetic measurements suggest long-range antiferromagnetic ordering with a Néel temperature (TN) of 4 K, which is corroborated by heat capacity measurements. The localized effective moment μeff (Ir) = 1.73 μB and insulating character indicate that Li8IrO6 is a correlated insulator. First-principles calculations support the nonpolar crystal structure and reveal the insulating behavior both in paramagnetic and antiferromagnetic states.
High-throughput search for magnetic topological materials using spin-orbit spillage, machine-learning and experiments
Physical Review B 103, 155131 (2021)
Preprint: arXiv:2102.00237
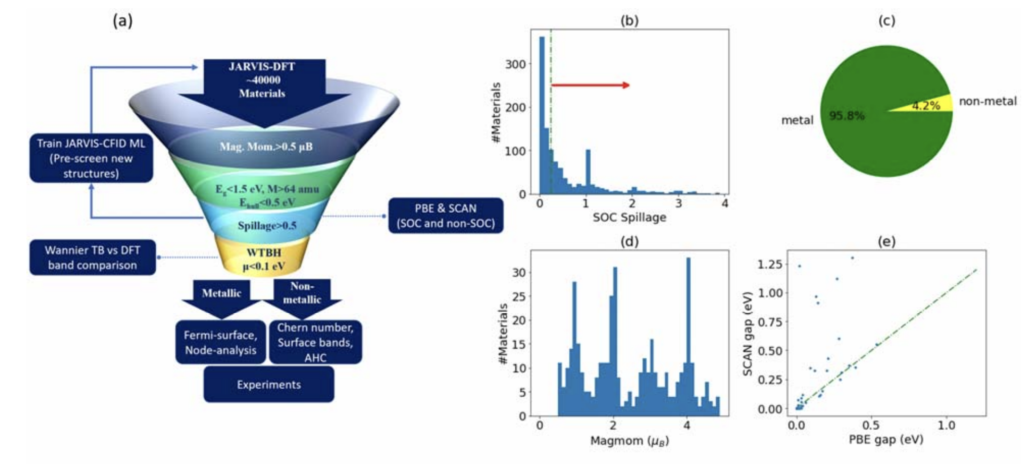
Magnetic topological insulators and semi-metals have a variety of properties that make them attractive for applications including spintronics and quantum computation, but very few high quality candidate materials are known. In this work, we use systematic high-throughput density functional theory calculations to identify magnetic topological materials from the ≈40000 three dimensional materials in the JARVIS-DFT database (https://jarvis.nist.gov/jarvisdft). First, we screen materials with net magnetic moment > 0.5 μB and spin-orbit spillage (SOS) > 0.25, resulting in 25 insulating and 564 metallic candidates. The SOS acts as a signature of spin-orbit induced band-inversion. Then, we carry out calculations of Wannier charge centers, Chern numbers, anomalous Hall conductivities, surface band structures, and Fermi-surfaces to determine interesting topological characteristics of the screened compounds. We also train machine learning models for predicting the spillages, band gaps, and magnetic moments of new compounds, to further accelerate the screening process. We experimentally synthesize and characterize a few candidate materials to support our theoretical predictions.
Isotropic Nature of the Metallic Kagome Ferromagnet Fe3Sn2 at High Temperatures
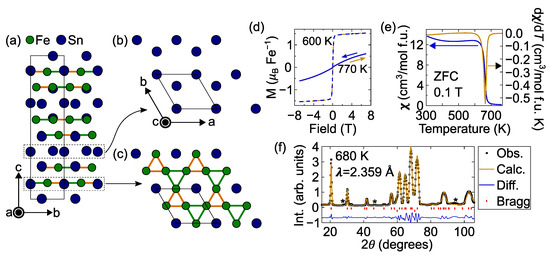
Anisotropy and competing exchange interactions have emerged as two central ingredients needed for centrosymmetric materials to exhibit topological spin textures. Fe3Sn2 is thought to have these ingredients as well, as it has recently been discovered to host room temperature skyrmionic bubbles with an accompanying topological Hall effect. We present small-angle inelastic neutron scattering measurements that unambiguously show that Fe3Sn2 is an isotropic ferromagnet below TC ≈ 660 K to at least 480 K—the lower temperature threshold of our experimental configuration. Fe3Sn2 is known to have competing magnetic exchange interactions, correlated electron behavior, weak magnetocrystalline anisotropy, and lattice (spatial) anisotropy; all of these features are thought to play a role in stabilizing skyrmions in centrosymmetric systems. Our results reveal that at the elevated temperatures measured, there is an absence of significant magnetocrystalline anisotropy and that the system behaves as a nearly ideal isotropic exchange interaction ferromagnet, with a spin stiffness D(T = 480 K) = 168 meV Å2, which extrapolates to a ground state spin stiffness D(T = 0 K) = 231 meV Å2.
Chiral properties of the zero-field spiral state and field-induced magnetic phases of the itinerant kagome metal YMn6Sn6
Physical Review B, 103, 094413 (2021)
Preprint: arXiv:2012.13010

Applying a magnetic field in the hexagonal plane of YMn6Sn6 leads to a complex magnetic phase diagram of commensurate and incommensurate phases, one of which coexists with the topological Hall effect (THE) generated by a unique fluctuation-driven mechanism. Using unpolarized neutron diffraction, we report on the solved magnetic structure for two previously identified, but unknown, commensurate phases. These include a low-temperature, high-field fan-like phase and a room- temperature, low-field canted antiferromagnetic phase. An intermediate incommensurate phase between the fan-like and forced ferromagnetic phases is also identified as the last known phase of the in-plane field-temperature diagram. Additional characterization using synchrotron powder diffraction reveals extremely high-quality, single-phase crystals, which suggests that the presence of two incommensurate magnetic structures throughout much of the phase diagram is an intrinsic property of the system. Interestingly, polarized neutron diffraction shows that the centrosymmetric system hosts preferential chirality in the zero-field double-flat-spiral phase, which, along with the THE, is a topologically non-trivial characteristic.
Competing magnetic phases and fluctuation-driven scalar spin chirality in the kagome metal YMn6Sn6
Science Advances 6, eabe2680 (2020)
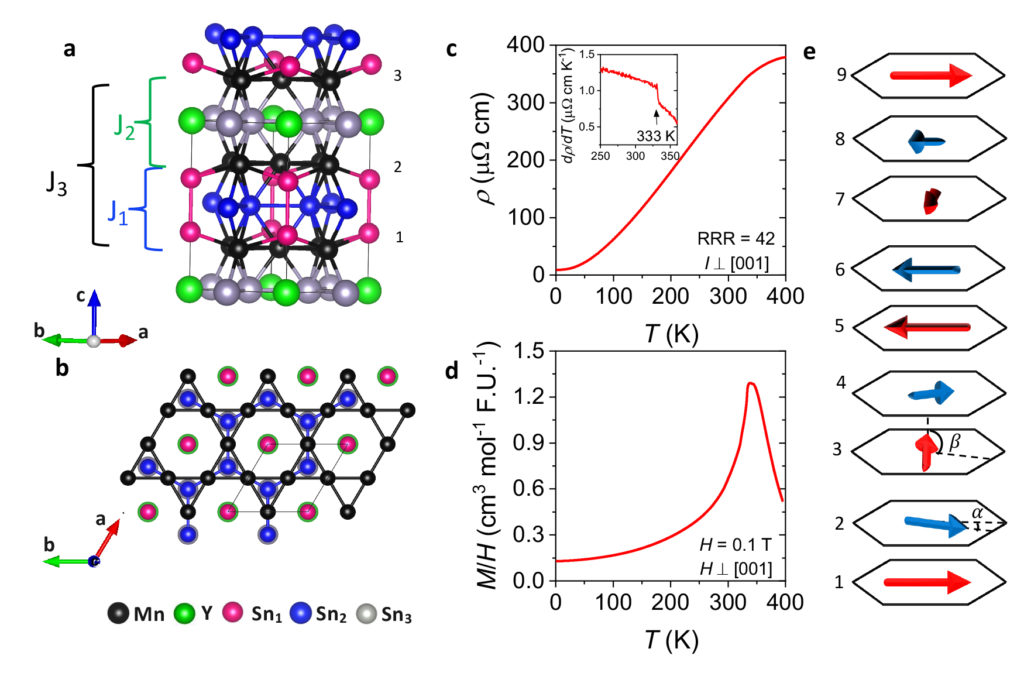
Identification, understanding, and manipulation of novel magnetic textures are essential for the discovery of new quantum materials for future spin-based electronic devices. In particular, materials that manifest a large response to external stimuli such as a magnetic field are subject to intense investigation. Here, we study the kagome-net magnet YMn6Sn6 by magnetometry, transport, and neutron diffraction measurements combined with first-principles calculations. We identify a number of nontrivial magnetic phases, explain their microscopic nature, and demonstrate that one of them hosts a large topological Hall effect (THE). We propose a previously unidentified fluctuation-driven mechanism, which leads to the THE at elevated temperatures. This interesting physics comes from parametrically frustrated interplanar exchange interactions that trigger strong magnetic fluctuations. Our results pave a path to chiral spin textures, promising for novel spintronics.
Fermi surface topology and non-trivial Berry phase in the flat-band semimetal Pd3Pb
Physical Review B 101, 245113 (2020)
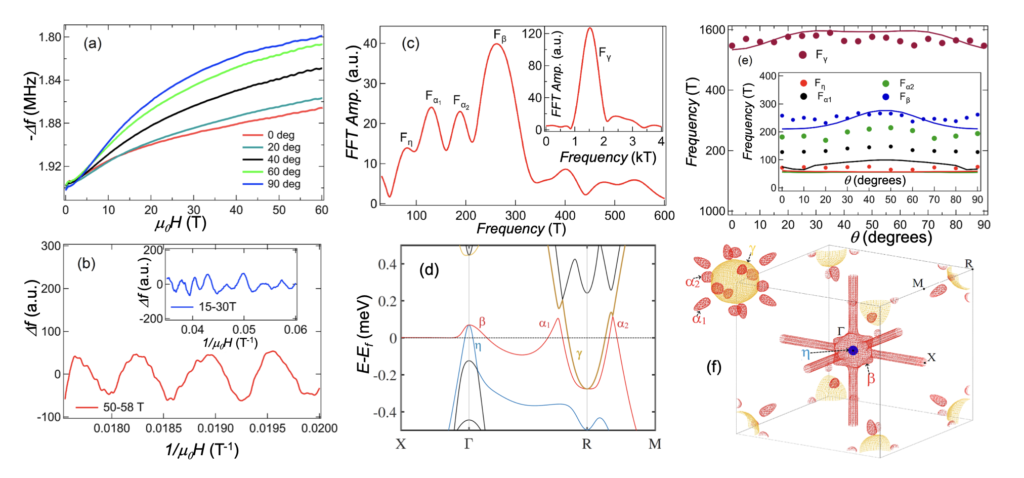
A study of the Fermi surface of the putative topological semimetal Pd3Pb has been carried out using Shubnikov-de Haas (SdH) oscillations measured in fields of up to 60 T. Pd3Pb has garnered attention in the community due to a peculiar Fermi surface that has been proposed theoretically by Ahn, Pickett, and Lee, [Phys. Rev. B 98, 035130 (2018)] to host a dispersion-less band along X−Γ as well as multiple triply-degenerate band crossings that, under the influence of spin-orbit coupling, lead to ten four-fold degenerate Dirac points. Analysis of the SdH oscillation data verifies the calculated multi-sheet Fermi surface, revealing a Γ centered spheroid that had not been resolved experimentally in prior studies. A comprehensive, angle-dependent analysis of the phase of the SdH oscillations convincingly demonstrates a non-trivial Berry phase for two bands along Γ − R, supporting the theoretical predictions, while simultaneously evidencing interference between extremal orbits that mimics a trivial Berry phase at intermediate angles.
Giant anomalous Hall effect in quasi two-dimensional layered antiferromagnet Co1/3NbS2
Physical Review Research 2, 023051 (2020)

The discovery of the anomalous Hall effect (AHE) in bulk metallic antiferromages (AFMs) motivates the search of the same phenomenon in two-dimensional (2D) systems, where a quantized anomalous Hall conductance can, in principle, be observed. Here we present experiments on microfabricated devices based on Co1/3NbS2, a layered AFM that was recently found to exhibit AHE in bulk crystals below the Néel temperatureTN =29K.Transport measurements reveal a pronounced resistivity anisotropy, indicating that upon lowering temperature the electronic coupling between individual atomic layers is increasingly suppressed. The experiments also show an extremely large anomalous Hall conductivity of approximately 400 S/cm, more than one order of magnitude larger than in the bulk, which demonstrates the importance of studying the AHE in small exfoliated crystals, less affected by crystalline defects. Interestingly, the corresponding anomalous Hall conductance, when normalized to the number of contributing atomic planes, is ∼ 0.6 e2 /h per layer, approaching the value expected for the quantized anomalous Hall effect. The observed strong anisotropy of transport and the very large anomalous Hall conductance per layer make the properties of Co1/3NbS2 compatible with the presence of partially filled topologically nontrivial 2D bands originating from the magnetic superstructure of the antiferromagnetic state. Isolating atomically thin layers of this material and controlling their charge density may therefore provide a viable route to reveal the occurrence of the quantized AHE in a 2D AFM.