
I am an Assistant Professor of Physics at the University of Notre Dame and the Stavropoulos Center for Complex Quantum Matter. I use two-dimensional materials to study emergent quantum phenomena with multiple experimental approaches, including microscopic high-quality 2D crystal assembly, cryogenic near-field microscopy and quantum transport.
My research highlights include:
- Manipulation of strong electronic interactions in graphene-based moiré materials.
- Local photocurrent studies of strongly correlated phenomena in magic angle twisted bi/trilayer graphene heterostructures.
- Plasmon polaritons in strongly correlated environment.
- Studies of time-reversal and inversion symmetry breaking in moiré electronic flat bands.
- Revealing coexisting superconductors and orbital ferromagnetic states in the same moiré device for further studies of exotic quantum phenomena.
- Studies of pure spin transport in graphene antiferromagnetic insulators.
- Quantum Hall effect in multi-Dirac band electronic systems.
Moiré quantum materials:
Ever since the experimental isolation of a single layer of carbon atoms in 2004 (a. k. a. graphene), the field of two-dimensional materials was rapidly expanding. Historically, novel nanodevice fabrication methods greatly advanced the low-dimensional materials research. Recent improvements brought a completely new degree of freedom to the heterostructure fabrication allowing for a fine tuning of a twist angle between two-dimensional layers. In early 2018, a group of researchers from MIT managed to fabricate magic-angle twisted bilayer graphene devices (MATBG) with the angle misalignment between the adjacent graphene layers of only 1.1 degree. This very special angle allows the two graphenes’ Dirac bands to hybridize in a very peculiar way creating a pair of very flat electronic bands in the zero-energy limit, which host superconducting, ferromagnetic and correlated insulating states. Its striking resemblances with other high-temperature superconductors generated an intense wave of theoretical and experimental research and opened a new field named “twistronics”. Nowadays the properties of the 2D materials can be significantly modified by a simple misalignment between the adjacent 2D layer, which are extensively used to study strongly correlated phenomena (i. e. phenomena that emerge as a result of the enhanced electron-electron interactions).
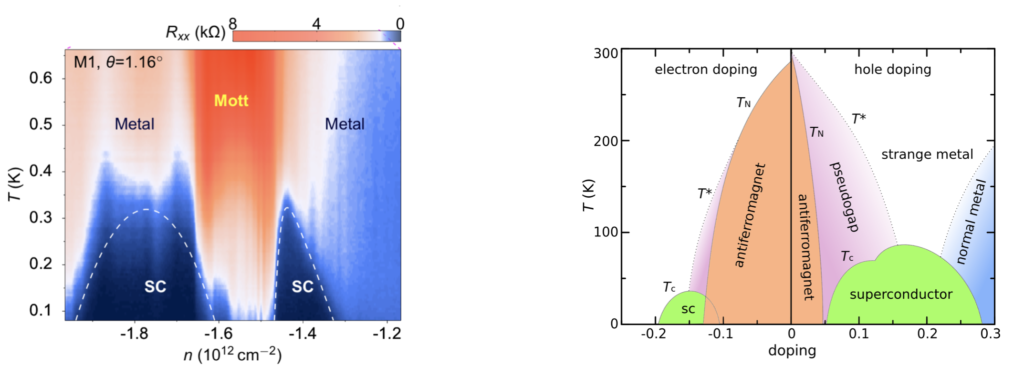
1. Scanning Probe Microscopy (SPM). Our current interest is to explore electronic interactions in moiré materials with a strongly confined light by using cryogenic near-field optical microscopy. Our preliminary results reveal interband plasmonic modes in twisted bilayer graphene devices. Many other interesting polaritonic modes are predicted in a number of moiré materials, which will remain the main focus of our research efforts. Full article [link].
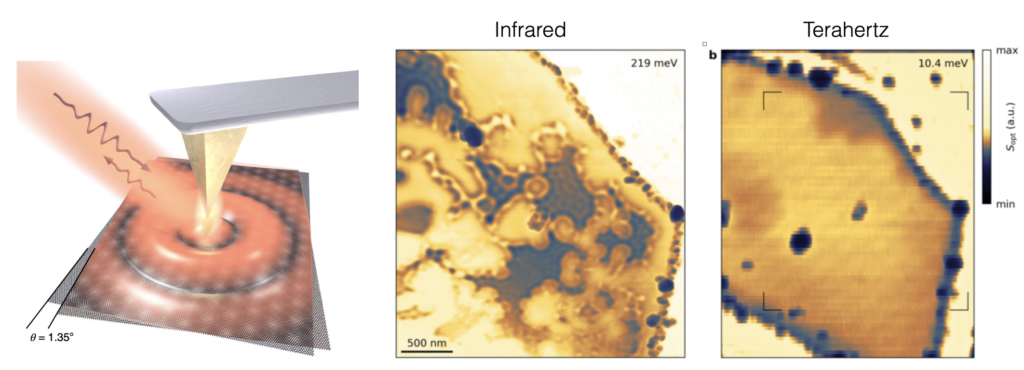
2. Quantum Transport and 2D Heterostructures. Tuneability of two-dimensional materials makes them an attractive candidate to study strongly correlated physics and attempt to learn something new about other strongly correlated systems. In one of our previous works we study the effect of electronic interactions screening in MATBG by proximitizing it to a closely located graphite back gate only a few nanometers away from the graphene plane. Turns out that in this case correlated insulators disappear from the phase space meaning that the superconductors in MATBG are not necessarily tied to them, unlike the case of high-temperature cuprate superconductors. Full article [link].
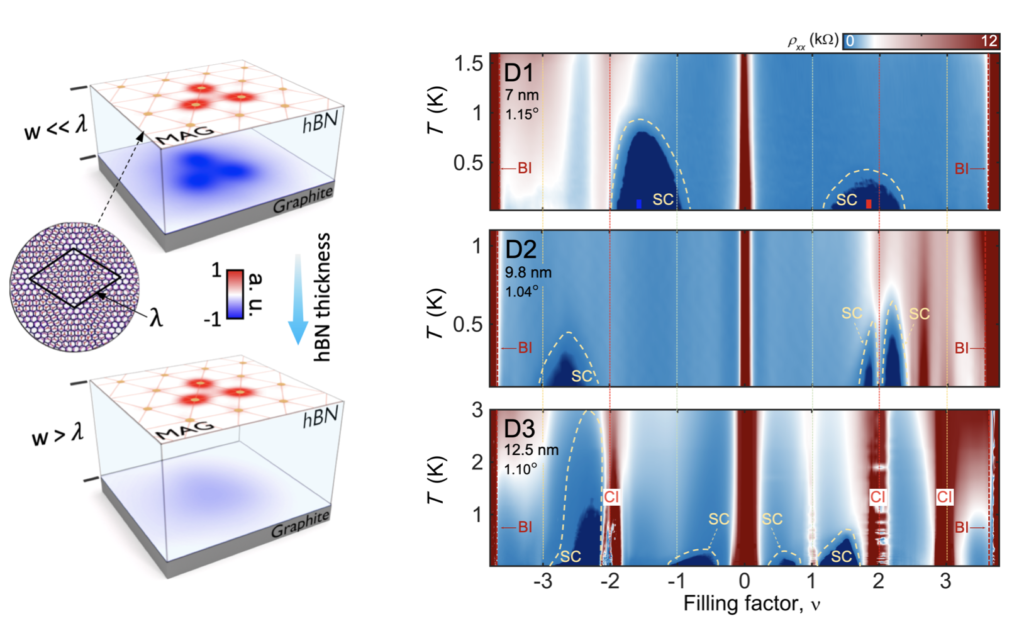
3. Complex Quantum Matter. In addition to superconductivity, strongly correlated electrons in MATBG may also show many other exotic states of matter, including orbital magnets and correlated insulators. In one of our previous works, we demonstrated an unprecedented tuneability of these states and studied their intricate nature in a MATBG sample, which showed both superconductivity and orbital ferromagnetism in the zero-magnetic field limit. Full article [link].
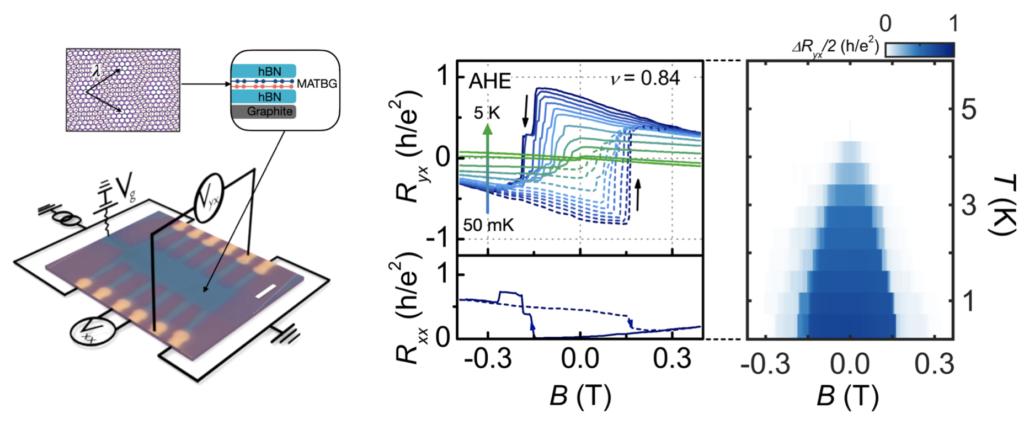