Parkinson’s disease is the second most common neurodegenerative disease with 90,000 Americans being diagnosed every year. Parkinson’s is a difficult disease to detect; there are no blood or lab test to diagnosis it. Diagnoses are symptom based. Parkinson’s patients often do not know they have the disease until after having dealt with it for years. Some of the early symptoms such as facial masking (lack of facial expression), small handwriting, or speaking softly do not directly point to a Parkinson’s disease diagnoses. A patient comes in with tremors, muscle rigidity, poor balance, and slowed movements and are then assumed to have Parkinson’s disease. If their symptoms improve with medication, the diagnosis is confirmed. Biomechanics is a useful tool in the evaluation of the progression of the disease as well as its continued treatment.
Continue reading “How is Biomechanics Used in the Treatment of Parkinson’s Disease?”Category: 2023 Fall
Footsteps and Force-Waves: New Methods of Measuring Lymphedema
The lymphatic system is a system of vessels and lymph nodes that runs parallel to our vascular system. It takes up extra fluid from around our cells, filters it, and returns the liquid to our circulatory system. When part of the lymphatic system is damaged by surgery, radiation treatments, or injury, a progressive disease called lymphedema can occur.
Continue reading “Footsteps and Force-Waves: New Methods of Measuring Lymphedema”How Your Brain Gets Rid of Junk By Force!
The human brain is an organ that scientists are always interested in due to its complexity. Insight on how to treat and/or cure physiological illnesses in the brain, such as Alzheimer’s disease or a stroke, could significantly improve the overall wellbeing of society. A new research area called brain lymphatic drainage has been able to demonstrate potential innovative therapeutic strategies to address these neurological diseases. A critical investigation of the brain biology along with biofluid flow by engineers has opened the door to another impressive brain process!
Read more: How Your Brain Gets Rid of Junk By Force!Brain Lymphatic Drainage
Humans have a lymphatic system in their body which is a system of lymphatic vessels that collect waste and other leaking fluids from blood vessels which helps maintain fluid levels in tissues. The lymphatic vessels deliver this fluid to lymph nodes, which are small organs containing immune cells, that filters and drains the fluid back to the blood.
The brain doesn’t contain lymphatic vessels and it was unclear how it drains its waste. However, many scientists and engineers have recently discovered that there are indeed components of a drainage system in the brain which is connected to the body’s lymphatic system. The interstitial fluid (fluid surrounding the cells within the brain that contain solutes; ISF) is sent to be mixed with cerebrospinal fluid (fluid between the brain and an outer dense tissue; CSF). This mixed CSF fluid is then drained into lymphatic nodes and is eventually returned to the lymphatic system. The collection and drainage of ISF or CSF can be done with multiple pathways (i.e., through outer tissue of brain, sponge-like bone near olfactory nerves, etc.). The figure below shows a simplified diagram of some brain lymphatic drainage pathways towards the lymphatic system.
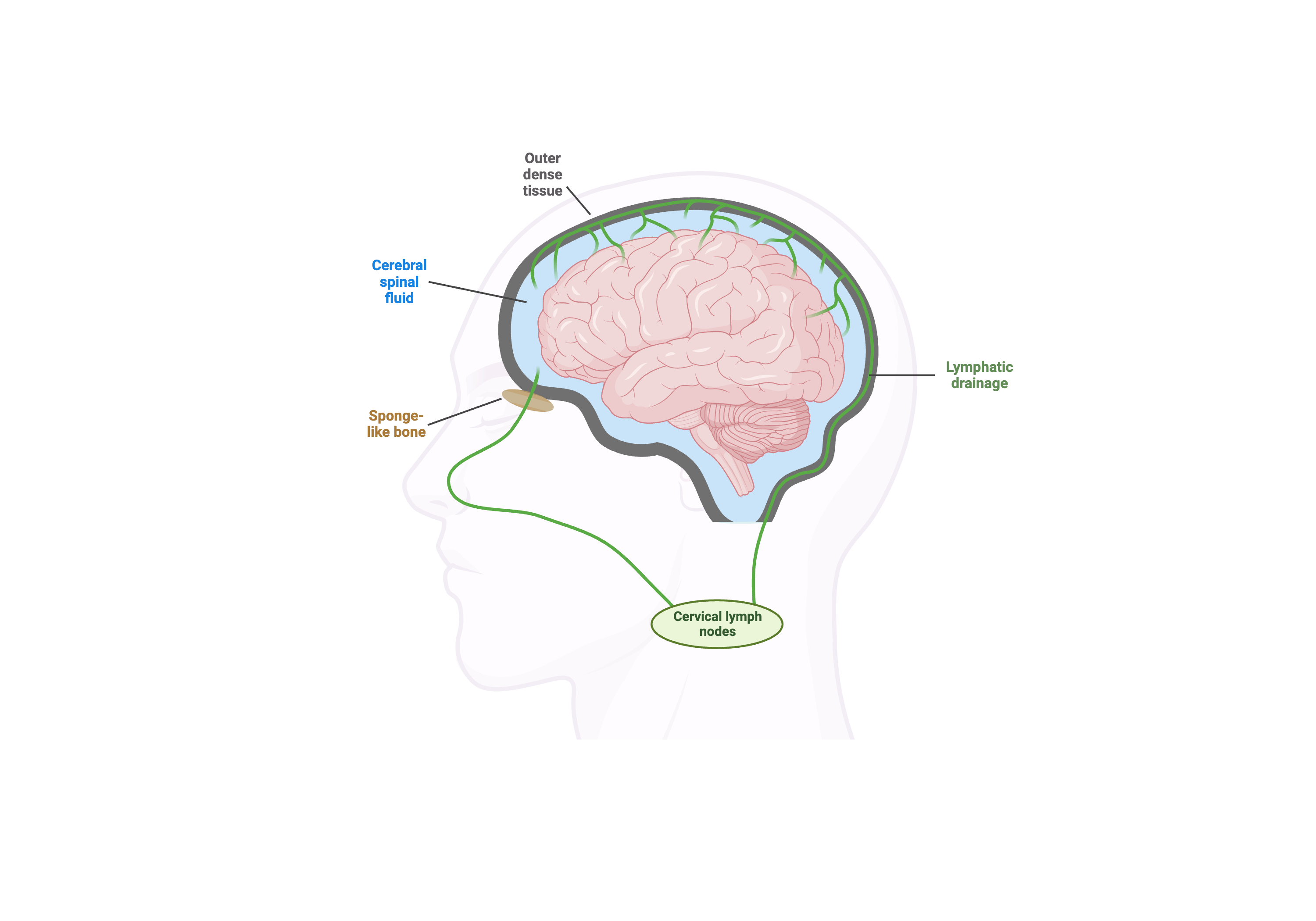
Fluid Dynamics in Brain Lymphatic Drainage
Fluid dynamics is the study of fluids in motion. In the proposed brain lymphatic drainage system, there is an exchange of ISF and CSF in fluid-filled compartments that surround the brain which is a vital process for maintaining a healthy brain. Thus, fluid dynamics can be used to describe the forces involved in driving this ISF-CSF exchange and its impact for brain health.
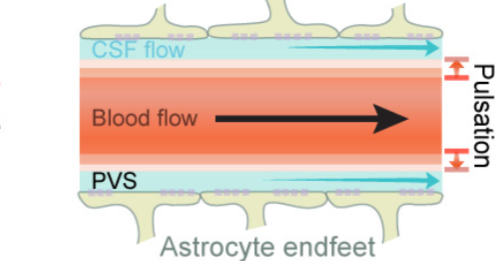
In a recent study, a group of scientists and engineers quantitatively investigated the flow of CSF in these fluid-filled compartments and identified it as a pulsatile flow (i.e., rhythmic and intermittent movement of fluid) driven by pressure changes within the heart. The above demonstrates how CSF flow is impacted. The authors then saw that an increase in overall blood pressure leads to a reduction of forward flow of CSF which can partly limit the ISF-CSF exchange and lead to accumulation of unwanted molecules in the brain. They stated that this would explain the previously identified correlation of hypertension and accumulation of peptides that are found in patients with Alzheimer’s.
Future Implications
The discovery of the brain lymphatic drainage system and how fluid dynamics plays a role has now allowed others to begin to explore new research areas. Some of these include repairing the sites of fluid flow disruption, taking advantage of the fluid flow routes to deliver drugs, and determining potential causes of neurological diseases (Alzheimer’s, multiple sclerosis, etc.) with respect to fluid drainage. However, there are many other factors within and surrounding the brain that impacts drainage that needs to be discovered to better understand brain lymphatic drainage.
Bend it like Beckham?: Genu Varum (Bow Leg) in Soccer Players
Soccer, or football for those outside of the United States of America, is the most popular sport in the world, and its popularity is only rising. In the United States, although soccer is not the most popular sport, it is growing rapidly. Part of the reason behind this growth, around 20% American parents think soccer to be a safer sport for their children to play compared to American football. However, soccer players still suffer injuries.
Continue reading “Bend it like Beckham?: Genu Varum (Bow Leg) in Soccer Players”Nature vs. Nurture: who is responsible for bone shape?
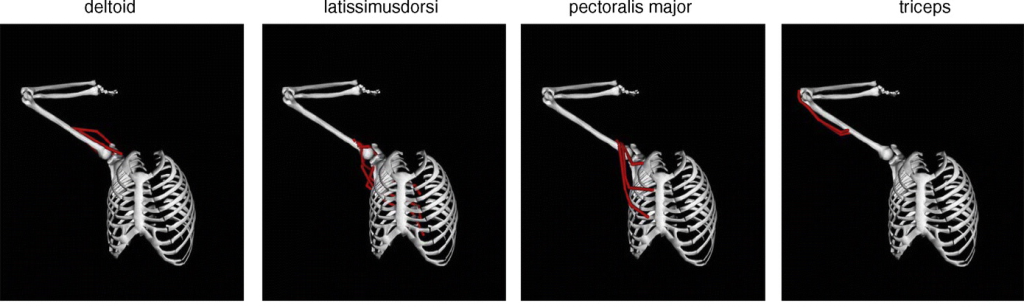
Bones are more than just spooky installments – they are the structural elements of the human body, like the steel girders of a skyscraper. They contain calcium-rich minerals and collagen fibers which are usually aligned along the long axis of the bone, known as the major axis. As a result, bones typically have material properties that are stronger in the axial direction. Nowadays, human bones can regularly experience forces much larger than loads that were experienced thousands of years ago. Especially in sports like powerlifting, these loads may be applied to bones in directions different than normally experienced during development. How does this affect bone structure in athletes today?
Continue reading “Nature vs. Nurture: who is responsible for bone shape?”You’re One Tough Nut To Crack!
On April 13, 2022, a video uploaded to YouTube titled The End broke the internet. A former Blue Sky Studios Employee, who over the years helped animate the Ice Age movie franchise, posted an unreleased short scene that depicted Scrat, the crazed squirrel from the series, finally catching and eating the acorn he spent more than a decade fighting and almost perishing over.
Continue reading “You’re One Tough Nut To Crack!”Avoiding Cat-astrophe: How do Cats Land their Crazy Jumps?
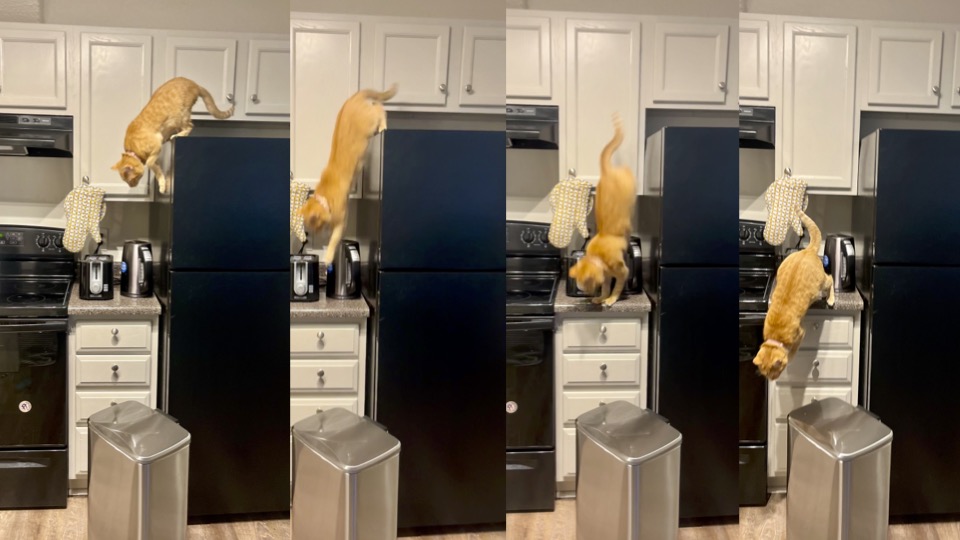
Cats always land on their feet, or so the saying goes. Every cat owner has witnessed their feline make death defying jumps and walk away like it’s no big deal. 90% of cats can actually survive falling off of a high rise building. But how do they do it? How do cats absorb the impact of their leaps without sustaining injuries?
Continue reading “Avoiding Cat-astrophe: How do Cats Land their Crazy Jumps?”A Sticky Situation: The Forest’s Tree-Climbing Superhero
As a child, did you ever watch with awe as Spider-Man climbed lofty buildings in New York City? This super-power has captivated audiences for decades because it seems impossible that a tiny fingertip could provide such great strength. However, the powerful and sticky toes of the tree frog bring this super-power to reality. Their toes have round pads that can cling to surfaces, both wet and dry, due to biomechanical forces. What causes their toes to be so sticky?
Read more: A Sticky Situation: The Forest’s Tree-Climbing Superhero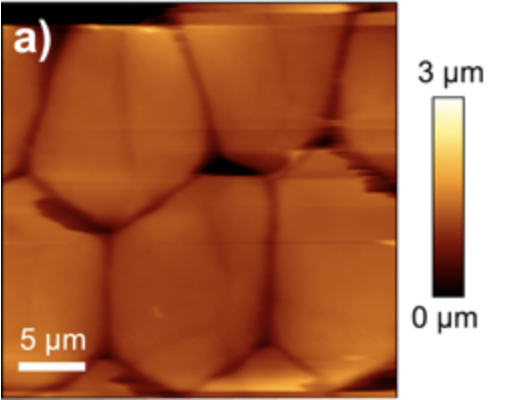
The round toe pads of the tree frog are composed of four layers of surface cells known as epithelial cells. Epithelial cells line all surfaces of the body, including the external surfaces and internal cavities of the body, such as the inner surfaces of organs. The epithelial cells of the toe pad are shaped like hexagons and columnar. Columnar cells have a greater height than width and resemble small pillars. These microscopic pillars are separated from each other by channels filled with mucous. This unique structure creates a phenomenon known as wet adhesion.
The word “adhesion” likely prompts thoughts of everyday adhesives like glue and tape. But, what is wet adhesion? The molecules in liquids are attracted to each other and to the surface surrounding the liquid. In a small container, these attractions cause what are known as capillary forces. When a straw is placed in water, liquid climbs the side of the straw due to these forces. Wet adhesion is the attachment of two materials due to the presence of a liquid with capillary forces. A good example of wet adhesion is when a piece of plastic that is wet gets stuck to a glass table.
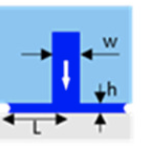
In the toe pads, the mucous secreted into pillar channels creates a layer of liquid between the pad and the external surface, which encourages capillary forces. The liquid layer has also been found to encourage viscous forces. Viscous forces are caused by the attraction between liquid molecules. The attractive forces resist the horizontal movement of liquid molecules when a force is applied tangent to the liquid’s surface. Capillary and viscous forces allow tree frogs to adhere to surfaces at steep angles without sliding off.
Recent research has also shown that frictional forces play a role in toe pad adhesion. The stiffness of the surface of toe pads is not uniform. The polygonal epithelial cells at the surface of the pad have a higher stiffness than the material below. The flexibility of the material below the surface allows for better contour of the pad to a surface. The enhanced interaction with the surface imperfections causes frictional forces to resist sliding and encourage adhesion.
The adhesive toe pads of tree frogs have inspired several engineering applications, including wet adhesive materials. Scientists are pursuing the fabrication of artificial materials that can mimic the characteristic micropillars of tree frog epithelial cells. For example, typical surgical tools, such as forceps, may struggle to effectively grasp softer tissues or increase the risk of tissue damage. Wet adhesive surgical graspers may provide a less abrasive method of holding soft tissues throughout a procedure. Tree frog-inspired wet adhesives may also be used to create sensors and monitors that can easily adhere to a patient’s skin. The study of wet adhesives has the potential to greatly benefit the medical field.
How Lower Body Mechanics Unlock Performance in the Pitching Delivery
Why can some pitchers throw 105 mph and some only 85? Baseball players are continuously trying to throw the ball faster and hit the ball further. The lower body muscles, especially the gluteus maximus/medius, adductors and and other pelvic movers, are essentially what power the throw and what can directly increase pitch velocity. Learning how to efficiently use the muscles in the lower body while pitching will allow players to optimize their performance, train correctly off the field, and prevent injuries.
Continue reading “How Lower Body Mechanics Unlock Performance in the Pitching Delivery”Pacific Rim In Real Life?
How Close are we to a Pacific Rim Reality?
Remember those giant hybrid kaiju-fighting robots from Pacific Rim where the brain of a kaiju (strange beast in Japanese) has successfully infected the mechanical brain of the robots and turned human’s greatest defense against them ? Well, it turns out, the boundary between science fiction and reality isn’t as far as we thought. A researched field “Necrobotics” has taken the world by storm and it is so new that Google is still highlighting “Necrobotics” as red. Imagine a world where nature’s most complex design is integrated into human’s innovation, leading to the most incredible biohybrid systems. If you are drawn the future application of this field or the potential harmony between biology and robotics, you’re in for a treat. In this blog post, we’ll be exploring the existing researches within Necrobotics and the future outlook on this unique field.
Necrobotics, a term derived from “Latinized form of Greek nekros” (relating to death ) and “robotics,” may sound a tad bit eerie, but it’s far from sinister. In fact, it’s all about bringing life to machines. The heart of the research is focused on producing biohybrid system that utilizes the intricate abilities of a living organism while combining with the precision and flawless decision making skills of a robot. Similar to our natural world, it draws inspiration from our environment such as the symbiotic relationship of Bees feeding on a flower’s nectar while carrying its pollen from plant to plant.
So, why should you be interested in this intersection of biology and technology? The applications are nothing short of astounding. One day, we will have biohybrid robots aiding in disaster relief events, enhancing our healthcare capabilities and assisting us in answering humankind most complex questions. These robots are able to mimic natural organism abilities, making them more adaptable, versatile, and resilient than conventional robots. From robotic limbs that respond to neural signals in the body to machines that slither like snakes, Necrobotics are in prime position to push humankind to the next level.
Scientists and engineers have developed a variety of technology by studying organisms that have evolved over millennia of evolution. These technology include surface wettability modification based on lotus leaves and Namib beetles, adhesion mechanisms that mimic gecko toes, and even sensing for smart materials by imitating the color-changing chameleon and the humidity-sensitive pine cone. In order to inform the design of robots and actuators, researchers have also taken inspiration from the locomotion of aquatic and terrestrial animals, such as starfish, jellyfish, and cephalopod. Here is a famous example of dead spider corpse used as a mechanical claw.
In conclusion, these scientific topics may have been initially perceived as science fiction but it has quickly garnered attention and are becoming a crucial step for mankind to take. Future discoveries in this field will have the potential to redefine countless industries while acknowledge nature’s design. So if you’ve ever imagined a time where science and nature coexist, now is the perfect time to get excited about necroboticsᅳthe future is here, and it’s amazing.
Read more: Pacific Rim In Real Life?