Band-Aids and stitches have been around forever. They are safe and common ways to address everything from paper cuts to deep wounds. However, these technologies each have their own drawbacks and researchers have looked to nature to improve upon them; enter the gecko, more specifically their feet. The goal of these scientists was to use the adhesive properties of a gecko’s foot pads as an alternative method to hold wounds closed, but how does this work?
What Makes Geckos so Clingy?
A gecko’s foot pads are unique instead of relying on any sticky substance, their toes have rows and rows of setae. Setae as you can see below are essentially rows of microscopic hooks that can cling in one direction. This causes no damage to the surface and because of the ways the setae are oriented, they are easy to remove from the surface in one direction. This is what allows a gecko to both cling to and easily remove their feet from a surface, or in our case makes adhesives made with this technique both secure and easier to remove than their counterparts.
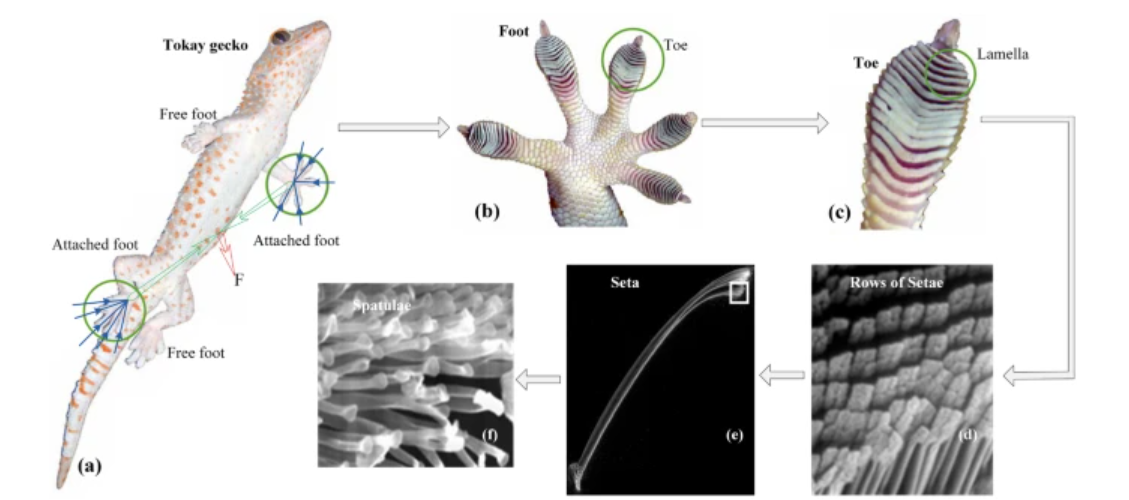
The Importance of Stickiness
Human skin is actually quite a rough surface that undergoes a lot of compression, tension and is further complicated by the existence of hair follicles and sweat. All of these factors make attaching anything like the gecko-based dry adhesive to the skin a daunting task. To get around this, bandaids rely on overpowering amounts of sticky substance and stitches are woven into the skin. This means that upon removal more damage and irritation to the wound can occurs. In a study the adhesive force of this technology was tested as well as the removal difficulty. It was found that this new method can achieve maximum closing forces slightly below that of sutures and is easier to remove than a bandaid of the same size. Notably, it does perform worse in wet or very humid conditions, so waterproof band-aids have a bright future.
The Design
A team of researchers published a study outlining the design, benefits, and drawbacks of a medical adhesive designed to mimic the gecko. This design encompasses the wound while providing a closing force to keep the wound closed. The amount of closing force imparted can be altered by changing both the number of legs and their thickness.
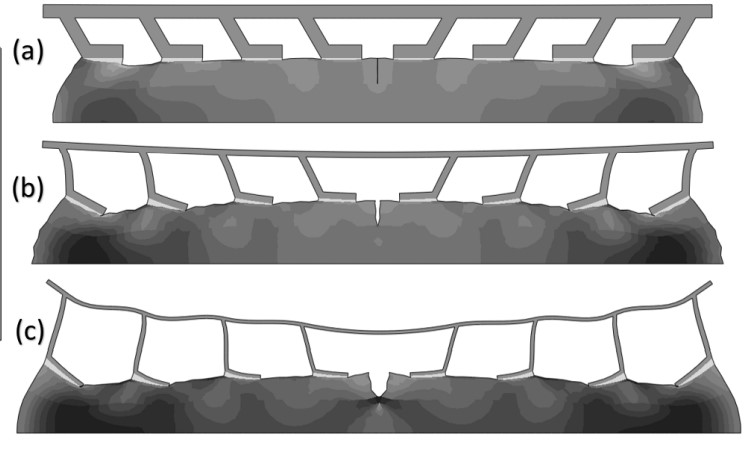
This is important because it means this method can be adapted to match the type of wound it is addressing. For a small cut a small adhesive with few legs can be used whereas, for a more serious wound, a larger adhesive layer with more, thicker legs will be used. This method also allows for air circulation allowing oxygen to reach to wound which promotes healing better than traditional methods. Using a gecko adhesive also minimizes wound irritation which can be a problem, especially for stitches and sutures which often cause irritation and even infection. Finally, this dry adhesive surface is biocompatible and can be made biodegradable. This means they will not react negatively with the skin and can safely dissolve in the body. This makes it a very desirable alternative to sutures which must be removed after insertion causing further wound irritation and requiring another visit to the doctor.
So while it may take a few years still don’t be surprised if you see dry adhesives for wound closings popping up on shelves; and when you see them know that they were inspired by the gecko. If you would like to learn more keep reading here.